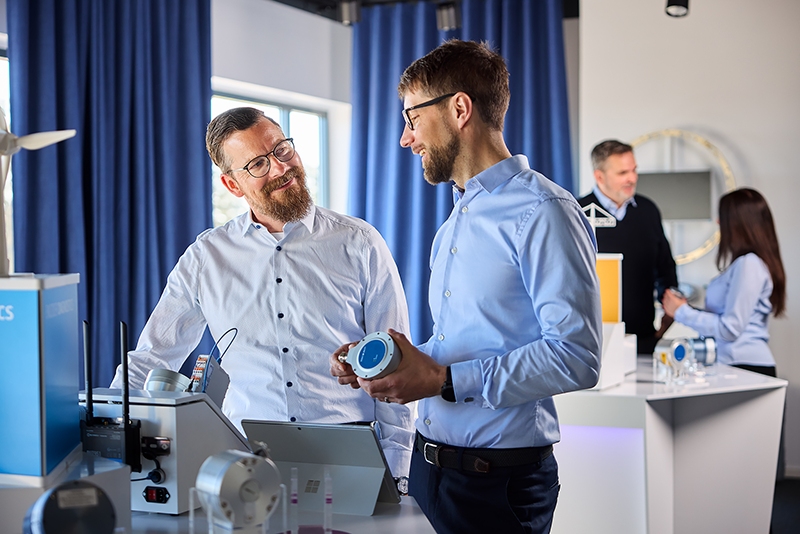
At Leine Linde, we take great pride in giving you personal support. You will get assistance and technical support throughout the product's lifecycle, from identification of your needs to delivery, installation, and encoder use or replacement. Maintenance questions? All questions are welcome! We work worldwide.
On time delivery
Production at Leine Linde is customer-order-driven, with the regular lead time as short as ten working days. Every encoder is traceable from customer order through manufacturing, tests and verification. The result is extreme accuracy and delivery performance.
24 hours manufacturing
Rapid manufacturing, also of customer-adapted products, is a question of honour to us. If the delivery is urgent, we offer 24 hours express manufacturing for most products.
48 hours manufacturing – On products requiring a 24-hour hardening process
Some products require a curing process of 24 hours to achieve the correct component endurance. We express manufacture such products in 48 hours.
Downloads
In the download section you will find brochures with technical data, mounting instructions, manuals, software and much more.
Warranty & Repairs
In the unlikely event that we fail at being flawless, you might want to read up on our warranty and repairs.
Track your order
Frequently Asked Questions / Tech Corner
-
An encoder can be either incremental or absolute.
Incremental encoders – for speed feedback or relative positioning
An incremental encoder usually generates a series of pulses in response to a linear or rotary motion. These pulses can be used to measure speed or be fed to a PLC or counter to keep track of a relative position. The output signal of an incremental encoder is normally an electrical square wave signal with a certain frequency related to the speed of the encoder shaft.
Absolute encoders – for positioning or digital speed
Absolute rotary encoders generate a position value that indicates the actual position of the encoder shaft directly. A major benefit of absolute encoders is that if the application loses power, the encoder is able to keep track of its position also if the shaft is turned during the power loss. This is due to the genuine absolute scanning principle. An absolute encoder can also be used to calculate a digital speed value. By internally dividing the difference in position with a small delta time an accurate speed value can be calculated and transmitted to the subsequent electronics.
Other types of encoders such as tachometers, i.e. an encoder with analog current outputs (0-20 mA or 4-20 mA) related to the speed or position of its shaft, may also be offered from Leine Linde. Thus the principle function of the encoder is always the same: an encoder converts a mechanical movement of its shaft into an electrical measurable unit representing the shaft’s speed or position.
Encoders are often used on electrical motors in the paper and steel industries, cranes and material handling systems as well as various types of measurement, testing and inspection systems.
-
Incremental encoders measuring principle
With the incremental measuring principle, the graduation consists of a periodic grating structure producing a defined number of sinusodial signals when the encoder shaft is rotated. These sinusodial signals can be converted into other signal formats and used in two different ways. Either for relative positioning or more commonly as speed feedback devices. Relative position information can be obtained by counting the individual increments (measuring steps) from some point of origin. When such a semi-absolute reference is required to ascertain positions, the graduated discs are provided with an additional track that bears a reference mark.
Image text: Incremental graduated code disc.
The rotational speed of the encoder shaft can be determined by calculating the frequency of the sinus-odial signals. Incremental encoders are normally used in closed loop, speed control loops or as speed feedback devices.
Resolution, line count and pulse rate
The resolution, line count or pulse rate are just different designations of the number of signal periods per channel and per revolution of an incremental encoder. Denominations of these signals vary between encoder manufacturers but Leine Linde commonly use S00, S90 and Sref. The signals S00 and S90 are 90 el° displaced from each other. S00 appear 90 el° before S90 when the encoder shaft is turned clockwise. A, B and N or K1, K2 and K0 are other examples of denominations used on incremental signals.
Image text: Output signals from an incremental encoder.For absolute encoders, resolution is stated as the number of bits. The number of bits (or unique positions per revolution) is calculated as 2n where n equals the number of bits. The total resolution of multiturn encoders also includes the number of distinguishable revolutions.
Measuring steps
In order to obtain higher resolutions from an incremental encoder, evaluation of all raising and falling pulse edges may be monitored. This is normally done by subsequent electronics as a quadruple, double or single evaluation. A measuring step is the definition of maximal number of edges as acquired when the subsequent electronics support quadruple evaluation, i.e. maximal measuring steps = 4 x line count. The example below indicates what different measuring step evaluation results in as seen by the subsequent electronics. In the example a 1024 ppr line count disc is used.
Image text: Measuring steps.
AccuracyThe accuracy of measurements with encoders is mainly determined by:
- Directional deviation of the radial grating
- Eccentricity of the graduated disc to the bearing
- Radial deviation of the bearing
- Error resulting from the mechanical installation
- Interpolation error during signal processing in the integrated or external interpolation and digitizing electronics
When speaking about accuracy of incremental encoders, the unit el° (electrical degrees) is normally used. For one signal period of the output signal is the equivalent of 360 el°. One revolution of the encoder equals 360 * N el°, where N is equal to the number of lines on the graduated disc (ppr).
Incremental encoders from Leine & Linde have a maximal permissible accuracy of ±50 el° (dividing error) which means that each pulse edge of the encoder signal has a deviation from its theoretical angle position of a maximum of 50/N°. As an example, for an encoder with 5000 ppr, ±50 el° corresponds to 0.01° maximum mechanical angle deviation from the theoretical position for each of the 20,000 pulse edges. (The encoder’s highest resolution is in this case 360/(5000*4) or 0.018°).
The dividing error is always sinus-shaped. One half of the revolution the pulses will have a shorter signal period where as the signal period will be a little longer for the other half of the revolution. If an incremental encoder is used in a speed control loop and has a high dividing error, this may be seen as a speed ripple.
Image text: Dividing error of an incremental encoder.For absolute encoders the term accuracy relates to the deviation from the absolute encoders optimal theoretical position. The unit used for accuracy of an absolute encoder is LSB, Least Significant Bit. On an absolute encoder with 13 bit singleturn resolution (213 = 8192 position) the accuracy is ±1LSB which implies that the maximal mechanical angle deviation is:
360° / 8192 = ±0.04°
Accuracy and calibration charts for each delivered encoder can be provided upon request.
Channel separation on incremental encoders
The specification of accuracy also includes the term channel separation, which is the distance between adjacent pulse edges of the S00 and S90 output signals. During final adjustments this is tuned to 90 el° and should lie within 90 ±25 el° for standard encoders. This means that the distance between adjacent pulse edges can vary between 65 el° and 115 el° for an approved encoder. Channel separation error is included in the dividing error.
The duty cycles of all incremental encoders are 180 el° or 1:1 unless stated otherwise.
Every delivered encoder is verified with respect to their accuracy, channel separation and duty cycle accomplished by monitoring that all pulse edges lies within approved limits. The measured values for maximum deviations from the encoder specifications are referred to the encoder’s serial number and collected in a database for statistical follow-up and future reference.
All accuracy data refer to measuring signals at an ambient temperature of 20°C, and with controlled subsequent electronics and transmission lines.
-
TTL electronics/RS422
Incremental TTL-signals are transmitted as digital squarewave pulse trains S00 and S90, phase-shifted by 90 el°. The reference mark signal consists of one reference pulse denoted as Sref, which is gated with the incremental signals. As an option on TTL-encoders, the integrated electronics also produce inverse signals of S00 and S90 for noiseproof differential transmission. In this case the encoder signals comply with the RS422 standard.
Image text: Output signals, TTL electronics.Interface Square-wave TTL or
RS422 (differential)Incremental signals S00, S90 (optional S00, S90) Reference mark
Pulse width
Delay timeSref (optional Sref)
90 el° (other on request)
td < 50 nsSTATUS (optional)
Pulse widthImproper function: Low
Proper function: High
ts > 20 µsSignal level Uh > 3 V with - Ih = 10 mA
Ul < 0.4 V with Il = 10 mAPermissible load Z0 = 100 W
Il < ±20 mA (per output)
Cload < 1000 pF
Outputs are short-circuit protected against 0V and +EVSwitching times
(10% to 90%)t+/t- < 200 ns
With 1 m cable and recommended input circuitry
Image text: Recommended subsequent electronics, TTL / RS422.The permissible cable length for transmission of the TTL square-wave signals to the subsequent electronics depends on the edge separation and whether differential (6 channels) or single-ended transmission is used. Note that the permissible cable length is calculated as long as the power supply can be ensured at the encoder. Make sure to compensate for voltage drop in the power supply lines.
HTL and HCHTL electronics
Leine Linde encoders with HTL interface incorporate electronics that digitize sinusoidal scanning signals. The incremental signals are transmitted as digital square-wave pulse trains S00 and S90, phase-shifted by 90 el°. The reference mark signal consists of one reference pulse Sref, which is gated with the incremental signals. In addition, the integrated electronics produce inverse signals of S00 and S90 for noise proof differential transmission. The fault-detection signal STATUS indicates fault conditions such as under voltage of the power supply or failure of the light source. It can be used for such purposes as machine shutoff during automated production.
To prevent counting error, the subsequent electronics should be designed to process as little as 90% of the edge separation a. See diagram below.
The permissible cable length for incremental encoders with HTL signals depends on the scanning frequency, the effective power supply and the operating temperature of the encoder.
Image text: Output signals, HTL / HCHTL electronics.
Interface HTL or HCHTL Incremental signals S00, S90 (optional S00, S90) Reference mark
Pulse width
Delay timeSref (optional Sref)
90 el° (other on request)
td < 50 nsSTATUS (optional)
Pulse widthImproper function: Low
Proper function: High
ts > 20 µsSignal level
(at 24V feed)Uh > 21 V with - Ih = 20 mA
Ul < 2.8 V with Il = 20 mAPermissible load Z0 = ±40 mA
Il < ±100 mA (per output)
Cload < 10 nF
Outputs are short-circuit protected max. 1 min against 0V and +EVSwitching times
(10% to 90%)t+/t- < 200 ns
With 1 m cable and recommended input circuitry
Image text: Recommended subsequent electronics, HTL.
Image text: The graph shows the permissible cable length at various frequences for HTL encoders. -
Parallel
Parallel output provides an absolute position available simultaneously on the output. It may be provided as binary or transformed in gray code format. Gray code means a single-bit change between each position step, which can reduce transmission errors.
Parallel output encoders can also accept inputs, for example setting the counting direction.
The advantage of parallel output is that it is fast and all the data is available in real time, all the time.
Analog
The absolute position can also be represented as an analog current output. 0-20 mA or 4-20 mA for a full-scale output.
Image text: Output signal analog interface.
On special request an analogue output with a teach-in functionality can also be offered. The teach-in function implies that the encoders active angle can be configured at will. A maximal full-scale output 0 or 4-20 mA current value can therefore be distributed over the total measuring range.
BiLL (RS485 based)
BiLL is a bi-directional master/slave communication used on absolute encoders. The protocol can be used for RS485 transmission standards or for a multi-drop bus system using the RS485 standard. Data are sent in hexadecimal format and the addressed encoder answers only on a master request. The protocol includes position data in binary format, a checksum for transmission reliability, a hold command, a change of baud rate command and an error message.
Serial point-to-point
Serial transmission means that bit information is transmitted sequentially in the same pair of conductors rather than sending each bit in its own conductor as in parallel transmission.
One of the advantages of serial transmission is that installation costs less; fewer wires means less work and less documentation.
There are several more or less standardized methods for serial transmission of data, all with their advantages and disadvantages. The following is a short description of the most common serial standards used for communication with encoders.
EnDat 2.1
The EnDat 2.1 interface is a digital, bidirectional interface for encoders. It is capable of transmitting position values from absolute encoders, as well as reading and updating information stored in the encoder.
Thanks to the serial transmission method, only four signal lines are required. The absolute position data are transmitted in synchrony with the clock signal generated by the subsequent electronics. The type of transmission (position values, parameters, diagnostics, etc.) is selected by mode commands that the subsequent electronics send to the encoder.
Image text: Recommended subsequent electronics.
A clock pulse (CLOCK) is transmitted by the subsequent electronics to synchronize data transmission. When not transmitting, the clock signal defaults to HIGH.
One data packet is sent in synchrony per data transmission. The transmission cycle begins with the first falling clock edge. The measured values are saved and the position value calculated. After two clock pulses (2T), the subsequent electronics transmit the mode command “Encoder transmit position value”. After successful calculation of the absolute position value (tcal - see table), the start bit begins the data transmission from the encoder to the subsequent electronics.
Interface
Endat 2.1
Clock frequency fc
100 kHz ... 2 MHz
(optional up to 16 MHz)Calculation time for Position value
tcal
< 5 µs
Recovery time
tm
tr
10 to 30 µs
Max. 500 ns
The encoder then transmits the absolute position value, beginning with the LSB. Its length varies depending on which encoder is being used. The number of required clock pulses for transmission of a position value is saved in the parameters of the encoder manufacturer. The data transmission of the position value is completed with the Cyclic Redundancy Check (CRC).
Image text: Data transfer EnDat 2.1.
EnDat 2.1 encoders are available with incremental 1Vpp signals.
Note: Every Leine & Linde gateway for fieldbus communications communicates with the encoder via the EnDat 2.1 interface.
EnDat 2.2
With EnDat 2.2 it is possible to transfer additional data with the position value without sending a separate request for it. EnDat 2.2 is compatible with EnDat 2.1.
The extended EnDat interface version 2.2 is compatible in its communication, command set and time conditions with the previous version 2.1, but also offers significant advantages. It makes it possible, for example, to transfer what is termed “additional data” with the position value without sending a separate request for it. The interface protocol was expanded and the time conditions were optimized as follows:
- Increased clock frequency (CLOCK) 16 MHz
- Optimized calculating time, position value acquisition within 5 μs
- Minimized dead time (recovery time) 1.25 to 3.75 μs
- Expanded power supply range, UP = 3.6 to 5.25 V or 3.6 to 14 V at encoder
Without
delay compensationWith
delay compensationClock frequency fc
100 kHz ...
2 MHz100 kHz ... 16 MHz
Calculation time for Position value
tcalf
Parameter
tac
Typical of EnDat 2.2 encoders: < 5 µs
Max. 12 ms
Recovery time
tm
tR
tST
EnDat 2.1: 10 to 30 µs
EnDat 2.2: 10 to 30 µs or 1.25 to 3.75 us (fc > 1 MHz) (parameterizable)
Max. 500 ns
– 2 µs to 10 µs
Data delay time
tD
(0.2 + 0.01 x cable length in m) µs
Pulse width
tHI
tLO
0.2 to 10 µs
0.2 to 50 ms to 30 µs (with LC)
Pulse width fluctuation HIGH to LOW max. 10 %
EnDat 2.2 encoders from Leine Linde are available with incremental 1 Vpp signals.
SSI
SSI or Synchronous Serial Interface, is a digital point-to-point interface. It provides unidirectional communication at speeds up to 1.0 MHz by the use of only 4 wires.
The absolute position value, beginning with the most significant bit, is transferred over the data lines (DATA) in synchrony with a CLOCK signal from the control. The SSI standard data word length for singleturn absolute encoders is 13 bits, and for multi-turn absolute encoders 25 bits. The position value is transmitted in gray or binary code format.
Image text: Permissible cable length SSI.
In the quiescent state the clock and data lines are on high level. The current position value is stored on the first falling edge of the clock. The stored data is then clocked out on the first rising edge.
After transmission of a complete data word, the data line remains low for a period of time (t2) until the encoder is ready for interrogation of a new value. If another data output request (CLOCK) is received within this time, the same data will be output once again. If the data output is interrupted (CLOCK = high for t > t2), a new position value will be stored on the next falling edge of the clock, and on the subsequent rising edge clocked out to the subsequent electronics.
Image text: Data transfer SSI.
Interface
SSI
Clock frequency T
1 ... 10 µs
Calculation time for Position value
tcal
< 5 µs
Recovery time
t1
t2
n
0.4 µs
12 ... 30 µs
13 ... 25 bit
For the 600 series encoders, the following functions can be activated via the programming inputs of the interfaces by applying the input to a logic high level, i.e +EV:
Direction of rotation
Continuous application of a HIGH level reverses the direction of rotation for ascending position values.
Zero setting (setting position to zero)
Applying a positive edge (tmin > 1 μs) sets the current position to zero.
Image text: Recommended subsequent electronics.
Note: The programming inputs must always be terminated with a resistor (see input circuitry of the subsequent electronics).
The SSI interface is also available in combination with incremental 1 Vpp, HTL or RS422 signals on certain models.
-
PROFIBUS DP
PROFIBUS is a powerful and versatile 2-wire non-proprietary open fieldbus standard defined by several international standards such as EN 50170, IEC 61158 together with different device profiles.
The encoder device profiles for PROFIBUS-DPV0, DPV1 and DPV2 define the functionality of encoders connected to a PROFIBUS-DP bus. There are two encoder profiles available 3.062 and 3.162 defining the functionality of encoder for the different versions of PROFIBUS DP.
Image text: Network and configuration of PROFIBUS
Encoder Profile for DPV0, profile number 3.062
The operating functions in this profile are divided into two device classes. Class 1 encoders offer basic functions that all PROFIBUS-DP encoders must support. An encoder of class 1 can optionally support selected functions of class 2 but these functions must be implemented according to the profile.
Encoders of class 2 must support all functions of class 1 as well as the additional functionality of class 2.
Encoder Profile for DPV1 and DPV2, profile number 3.162
In addition to the functionality enabled in DPV0 and acyclic data exchange, expansions to the PROFIBUS were required to enable the interface in time-critical applications. As a result, DP-V2 functionality such as slave-to-slave communication and isosynchronous data exchange was added.
Slave-to-slave communication means, as the name implies, that slaves in a net can exchange information with each other via broadcast messages without communication being initiated by the master. This type of communication is very efficient and fast, which reduces the response time on the bus by up to 90%.
Isosynchronous data exchange implies that the master can reach several slaves simultaneously with for example set point values, or receives feedback values from different slaves. With the isosynchronous mode, a system can be set up where all slaves set their output values and read their input values at the same time with a very high accuracy. This functionally results in synchronization between many different slaves within 1 μs.
For further information regarding the Encoder functionality refer to the device profiles. These profiles and PROFIBUS technical information can be ordered at PNO in Karlsruhe, Germany (www.PROFIBUS.com).
To choose between the different profile versions, different GSD files are available. The user can select the version that fits their hardware and software.
Encoder functionality
The encoder can be configured as a class 1, 2 (DPV0) or class 3 or 4 (DPV2) PROFIBUS slave device. Class 2 configuration is extended to optionally access speed information from the encoder.
In the basic class 1 or 3 configuration only output values/positions are available.
The following functions can be performed or programmed:
- Position read out
- Changed direction of counting
- Diagnostic data up to octet 16
The following functions are available in addition on the class 2 or 4 functions:
- Scaling function
- Preset Value Function
- Speed read-out (class 2)
- Extended diagnostic data
PROFINET IO
ROFINET is an open standard for industrial Ethernet and uses TCP/IP and IT standards. This fieldbus interface satisfies all requirements for automation technology and it is widely used within factory automation and process automation. PROFINET IO describes an I/O data view of distributed I/O. It includes real-time (RT) communication and isochronous real-time (IRT) communication for cyclic process data. PROFINET is standardized in IEC 61158 and IEC 61784.
Leine & Linde PROFINET encoders conform to the encoder profile v4.1 (3.162) for PROFIBUS and PROFINET. The encoder profile version 4.1 is a further development of the encoder profile for DPV2 encoders’ version 3.1. It includes all the encoder functionality from encoder profile version 3.1 but it has been expanded with the usage of encoders with PROFINET and additionally the definition of a 64 bit position value.
Application class definition:
Class 3
Encoder with base-mode parameter access and limited parameterization of the encoder functionality. Isochronous mode is not supported.
Class 4
Encoder with scaling, preset, code sequence and base mode parameter access. Isochronous mode (IRT) is supported.
CANopen
The CANopen communication profile is based on the CAN Application Layer (CAL) specification from the CiA (CAN in Automation). CANopen is regarded as a robust fieldbus with highly flexible configuration possibilities. It is used in many various applications all based on different application profiles.
CANopen comprises a concept to configure and communicate real-time data using both synchronous and asynchronous messages. Four types of messages (objects) are distinguished:
- Administrative messages (Layer Management, Network Management, etc.)
- Service Data Messages (SDO)
- Process Data Messages (PDO)
- Pre-defined Messages (Synchronization-, Timestamp-, Emergency Messages)
For further information please view the CANopen specification available at www.can-cia.org.
Image text:Network and configuration of CAN
The Encoder Profile defines the functionality of encoders connected to CANopen. The operating functions are divided into two device classes:
Class 1
The Mandatory class with a basic range of functions that all encoders must support. The class 1 encoder can optionally support selected class 2 functions, these functions must however be implemented according to the profile.
Class 2
Where the encoder must support all class 1 functions and all functions defined in class 2. The full class 2 functionality includes:
- Absolute position value transfer using either polled, cyclic or sync mode
- Speed and acceleration output values
- Change of code sequence
- Preset value settings
- Scaling of the encoder resolution
Advanced diagnostics including:
- Encoder identification
- Operating status
- Operating time
- Alarms and warnings
All programming and diagnostic parameters are accessible through SDO’s. The output position value from the encoder is presented in a binary format.
DeviceNet
DeviceNet is a low-level network that provides connections between simple industrial devices (sensors, actuators) and higher-level devices (controllers). DeviceNet provides Master/Slave and Peer-to-Peer capabilities over the CAN bus.
DeviceNet has two primary purposes:
- Transport of control-oriented information associated with low-level devices
- Transport of other information, which is indirectly related to the system being controlled, such as configuration parameters
A DeviceNet node is modelled as a collection of Objects. An Object provides an abstract representation of a particular component within a product. The realization of this abstract object model within a product is implementation-dependent. In other words, a product internally maps this object model in a fashion specific to its implementation.
Like all other fieldbus interfaces, there is also an Encoder Profile which defines the functionality of encoders connected to a DeviceNet network. In the Encoder Profile are all Objects described that are used from DeviceNet Object library. Particular interesting is the Position Sensor Object (0x23 Hex). It describes the services that are available for fetching positions, scaling of position values and other useful information.
The full profile describes the encoder functionality which includes:
- Absolute position value transfer
- Speed output values
- Change of code sequence
- Preset value settings
- Scaling of the encoder resolution
Advanced diagnostics including:
- Encoder identification
- Operating status
- Operating time
- Alarms and warnings
DRIVE-CLiQ
DRIVE-CLiQ is an Ethernet-based communication protocol from Siemens for connection of sensors. This interface is specially made for drive applications for an easy connection between components such as converters, motors and sensors. With a speed of 100 Mbit/s and a cycle time of 31.25 µs, DRIVE-CLiQ has the performance required for the most demanding applications. Components with DRIVE-CLiQ can be automatic configured with each other since every component has an electronic label to store component-specific data used during commissioning drive systems. Another characteristic with the protocol is that the cabling onsite is reduced. Up to five units may be connected to a hub for transfer of data over a common cable. The Drive-CLiQ encoders are designed for transfer of both speed and position and are supplied with specially adapted connectors, with power supply and data in the same connector making it easy to connect the encoders.
We are here for you
At Leine Linde, we take great pride in giving you personal support. You will get assistance and technical support throughout the product's lifecycle, from identification of the customer's needs to delivery, installation, and encoder use or replacement. Maintenance questions? All questions are welcome! We work worldwide.
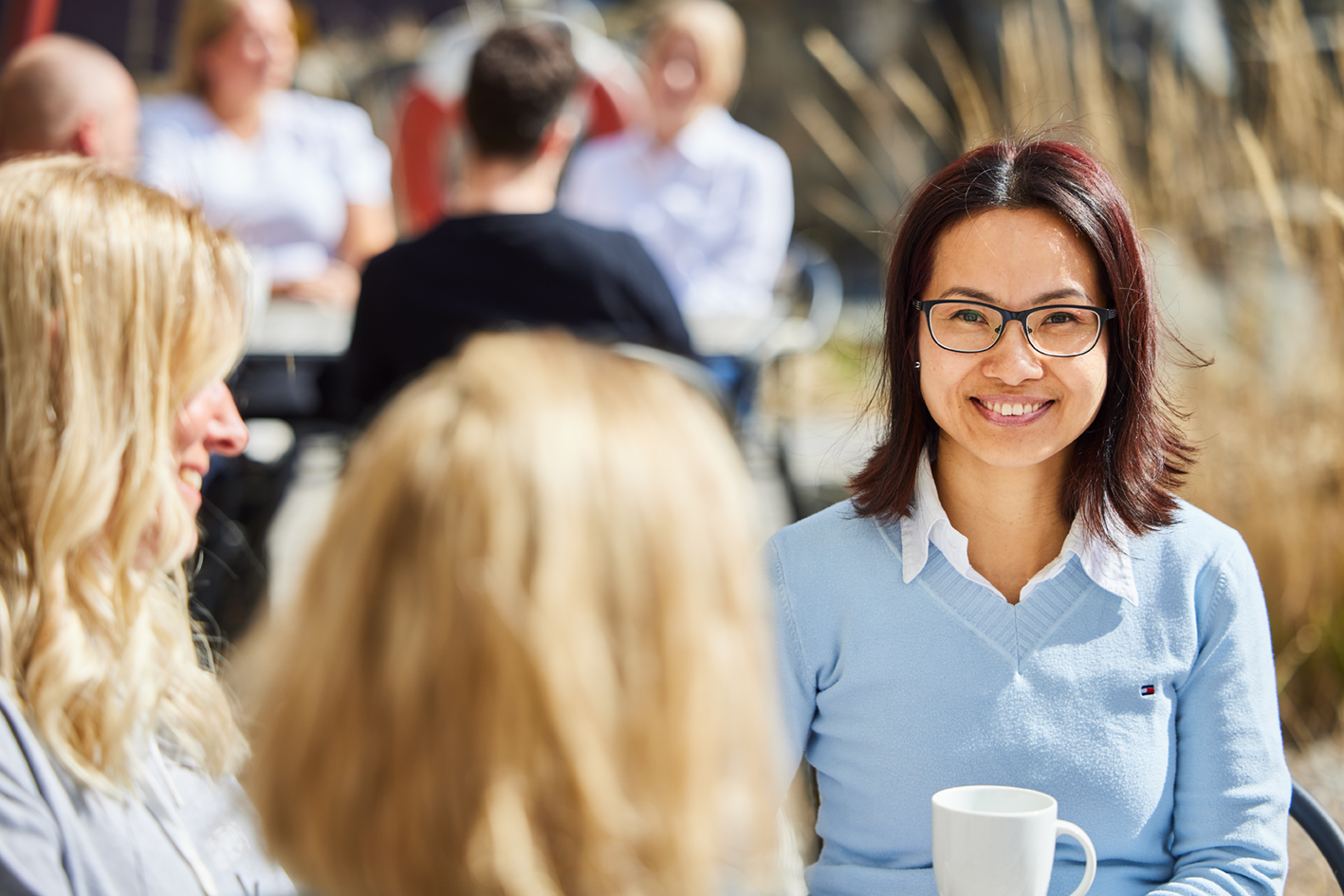